Shielding and Channelling
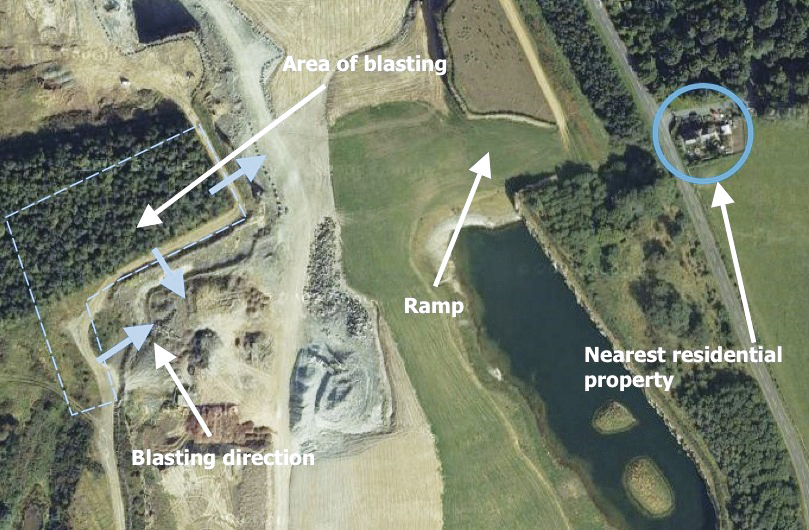
The influence of topography on air overpressure from quarry blasting
By Liam Bermingham, Blast Log Ltd
Air overpressure (AoP) is becoming an increasingly problematic issue within the quarrying industry. With blasting operations coming ever closer to residential properties, the number of complaints about air overpressure is increasing. It is important for operators to have an understanding of the causes of air overpressure and the factors that have an effect on air overpressure levels as they attenuate from a blast location. This article highlights how the topography surrounding a blast site has been shown to influence air overpressure travel paths and, ultimately, the magnitude of air overpressure experienced at nearby monitoring locations.
Shielding and channelling effects on air overpressure
The topography along the air overpressure travel path between the blast site and a monitoring location can provide a shielding effect, increasing the rate of attenuation. Where blasting takes place in deep excavations, hilly terrain or site barriers can partially obstruct the travel path of an overpressure wave, shielding some locations from the blast and resulting in lower levels of air overpressure at those locations. The relationship between the shielding effect, effective barrier heights and angle of incidence, shown schematically in figure 1, has been investigated and calculated by Richards and Moore (2002), but their work in figure 2 shows that a very large barrier height would be required to reduce AoP levels. For example, in figure 2 an effective barrier height of 40m and an incident angle of 40° should provide a reduction in AoP levels by the shielding of about 7dBL. Due to the low-frequency content of air overpressure, it is difficult to entirely shield an area that lies in the wave’s travel path.
While topography can shield air overpressure waves from a location, features such as valleys can channel the pressure wave, reducing the attenuation rate. The pressure wave can also become contained within a steep-sided valley, reflecting off the valley walls and reinforcing itself, potentially resulting increased AoP levels.
Case study on the channelling effect
Blast Log Ltd were asked by a quarry operator to monitor a series of blasts where high air overpressure levels were being recorded at a nearby residential property and to determine if and how the levels could be reduced. It was found that topographic features between the blast site and the property, created as part of the quarry’s restoration scheme, were playing a significant part in the problem.
Between the blasting area and the residential property a ramp is present (plates 1 & 2). This is not a natural topographic feature but was constructed as part of the final restoration scheme. The ramp is flanked on either side by near-vertical, c.10m high rock faces that reflect the pressure waves up the slope, channelling the air overpressure towards the property. This can increase the magnitude of the air overpressure as the reflected waves are superimposed on to each other.
Monitoring results
The AoP results recorded at known distances from a single-hole blast are shown in figure 3 and table 1. Figure 3 shows declining AoP levels from the nearest monitoring location to the blast, at a distance of 75.2m, to monitoring location 2. At distances beyond location 2 the data points circled in red clearly indicate that along the pressure wave travel path, the level of air overpressure increases as the distance increases from the blast, to monitoring location 4 positioned midway up the ramp. At monitoring location 5, at the top of the ramp in open terrain, the level reduces again. Table 1 shows a significant increase in levels from 130.6dBL at location 2 to 132.8dBL at location 4.
Predicted AoP levels without the channelling effect
In open terrain and without the influence of a channelling effect, the air overpressure in relation to distance is expected to decrease steadily away from the source. Figure 4 shows the monitored AoP levels shown in figure 3 against predicted AoP levels as if there were no channelling effect, generated using the power curve equation and values recorded in open terrain where no channelling effect occurred.
Figure 4 and the predicted and actual AoP levels in table 2 show the extent of the wave reinforcement along the ramp, with a difference of 4.3dBL at location 5, close to the nearest residential property. Reflection of the overpressure waves along the ramp, causing wave front reinforcement, is shown to result in a significant increase in levels.
Further evidence of the channelling effect
The single hole was loaded as a two-deck blast, but the AoP trace shown in figure 5 and recorded directly in front of the face at a distance of 75.2m shows only one AoP pulse. Video footage of the blast shows that the bottom deck of explosives did not project rock or cause the face to move. The blast did not misfire and the lack of face movement was thought to be due to the low charge weight used. Face movement was only produced from the top deck of explosives, hence the single AoP pulse.
The AoP trace shown in figure 6 was recorded at location 4 on the ramp and shows again the main single pulse as well as a second smaller pulse immediately after the higher-amplitude pulse. Monitoring locations in front of the blast in open terrain have shown that only one pulse was produced from the blast, whereas the monitoring locations along the ramp (flanked by the rock faces) show a second pulse. This is almost certainly due to the rock faces reflecting and reinforcing the pressure wave as they progress further up the slope, resulting in the second pulse. The wave trace recorded at the midpoint of the ramp clearly shows the impact of the rock faces and the reflection of the pressure wave. This effect will, consequently, produce higher amplitudes from a multi-hole production blast due to the reflected air overpressure pulse interacting with the initial positive phase within the pressure wave generated by the next hole in the firing sequence.
Channelling of air overpressure was also evident in multi-hole production blasts. Figure 7 and table 3 show that the AoP increased by 9.5Pa (3dBL) from the bottom of the ramp (location 5) to its midpoint (location 6) even though the distance from the blast had increased by 29.2m. Pressure levels at the top of the ramp (location 7) reached 31.5Pa, which is 7.5Pa (almost 2.5dBL) higher in magnitude than at the foot of the ramp even though monitoring unit 7 was 58.65m further away from the blast.
Conclusions
Increased AoP levels recorded on the ramp are apparently due to the channelling effect of the ramp and pressure waves reflecting off of high rock faces. To minimize this effect, changes to the orientation of blasting were recommended to avoid blasting where the face is orientated towards the ramp, as AoP travelling out directly in front of the face is always highest in terms of magnitude.
Other recommendations included changing aspects of the blast design, including inter-hole and inter-deck delay timings, in an attempt to reduce the reinforcing of pressure waves.
These findings show how influential topographic features can be in the attenuation of air overpressure waves generated from a blast. It is important, therefore, for blasting operators to take into consideration the direction in which the blast is orientated in relation to topographic features and sensitive locations. The location of nearby sensitive locations should also be considered when phasing quarry operations and progressive restoration schemes. For further information visit: www.blastlog.co.uk
References
RICHARDS & MOORE: Airblast Design Concepts in Open Pit Mines, Procs Fragblast 7 Conference, Beijing, August 2002, pp553-561.