Optimizing Fragmentation
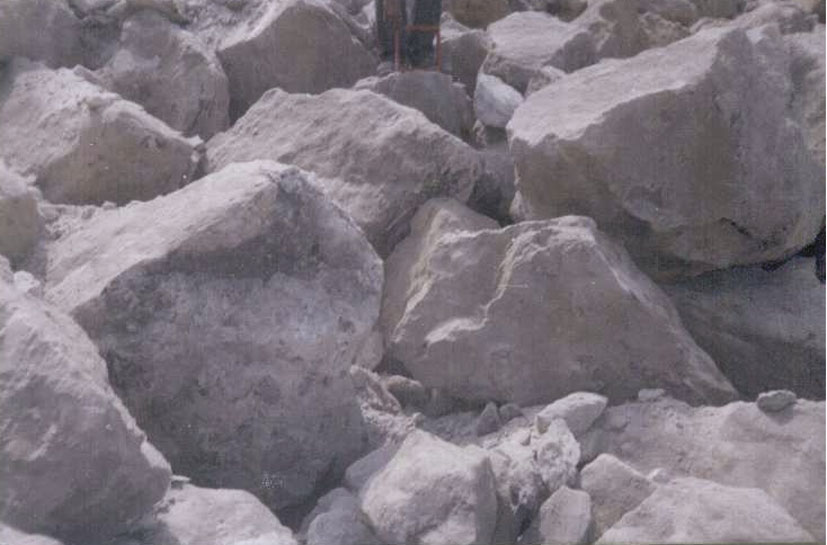
A study of the impact of stiffness ratio on the fragmentation of sandstone strata in an opencast coal mine
By Dr Piyush Rai*, Abhai Kumar Ranjan** and B. Singh***
The ratio of bench height (H) to effective firing burden (Be) represents the stiffness ratio in a surface mine bench blast. Changing the bench height, effective burden and/or the mode of firing may change this ratio in field-scale blasts. This is because, for a given burden, there exists a maximum bench height to produce a full crater. The breakage angle for a given burden increases with the increase in bench height up to a certain point. This phenomenon is attributable to the decrease in the burden rock stiffness, which makes the bench more flexible for improved fragmentation results. Lundberg et al.1 reported a host of problems due to increased burden rock stiffness.
The mechanism of rock fragmentation also considers the role of flexion in rock breakage during and after the radial fracturing and spalling phases; the pressure applied by the explosion gases in front of the explosive column acts on the burden rock mass, which behaves like a beam embedded in the bottom of the blasthole and in the stemming region. This beam is subject to breakage by deformation, fracturing and flexion, as depicted in figure 1.
The potential problems in relation to the changes in stiffness ratio are summarized in the table 1.
Sarathy4 proposed the concept of optimum bench heights for varying burdens with respect to the drill hole diameter, as given in table 2.
It is interesting to note that, even under similar bench-height conditions, blasts can be fired using different firing patterns that change the effective burden5, which changes the stiffness ratio and the stiffness of the bench. The role of effective burden when firing the blasts thus becomes consequential. Studies pertaining to the stiffness ratio can be made accordingly.
Objectives
The present study investigates the influence of stiffness ratio on the degree of fragmentation in field-scale blasts in order to establish a relationship between the fragment size and the stiffness ratio. To meet the above objective, blasts were conducted in one of the opencast projects of Northern Coalfields Ltd (NCL) in Singrauli, India.
The explosive and rock parameters were kept identical as far as possible in order to categorically assess the impact of stiffness ratio alone on the fragmentation results (under almost equivalent blast geometry parameters). In order to keep the rock parameters constant, all the blasts were conducted on the same bench with no (or insignificant) geological variations.
The studies were performed on a moderately strong sandstone overburden formation with compressive strength ranging from 10–25MPa and tensile strength from 0.8–2.0MPa. The sandstone was fine-to-medium grained. The coal in this particular coalfield is poor in grade and the mine is linked to a thermal power plant.
The explosive used in the Singrauli opencast coalfield was an emulsion comprising hydrated ammonium nitrate plus oil (burnt furnace oil, wax or paraffin), plus sodium nitrite as gassing agent, plus sodium mono-oleate as emulsifier, doped on site with 12–20% ammonium nitrate prills by site mixing trucks. This explosive was used throughout the entire project with no perceptible variations in its nature and properties, hence the explosive parameters have also been assumed to be identical for different blasts studied on the same overburden formation.
Field study and methodology
Four field-scale blasts were conducted and studied on the sandstone overburden bench. This bench was worked by a large-capacity shovel and dumptruck combination. Details of the four study blasts, with respect to blast geometry and other relevant parameters, are tabulated in table 3. As is evident from the table, the blasts were conducted at varying stiffness ratios. To measure the fragmentation in the blasted muckpiles, image capturing, processing and analysis techniques were employed. The imaging technique has been used with respect to blasting and fragmentation for the past two decades. This technique can precisely quantify the geometric aspects of the broken fragments using images in two dimensions6. The basis of this method is to capture scaled images of the blasted muckpile using a camera in the field, and then to digitize and measure the delineated fragments to provide a measure of the particle size distributions7,8.
In the present study, the blasted muckpile was documented with scaled photographs captured from the front of the blasted bench by keeping a suitable scale at physically convenient locations on the huge muckpiles. Obviously a single photo can not evaluate a large muckpile, hence a series of photographs was obtained periodically to cover the entire excavation sequence of the muckpile.
A number of additional photographs were captured in the event of exceptional field situations, such as the occurrence of large-size boulders or a large amount of fines, evidence of geological features etc. Typically 200–250 broken rock fragments could be easily captured in one image frame. Analysis of 20–25 photographs would yield a statistically representative sample for characterizing the fragmentation in one blasted muckpile. This is as per the recommendations of Paley9, Exadyktlos and Tsourtrelis10, Panlagio and Franklin11 and Scott et al.12.
For the purposes of processing and analysing the captured images, Fragalyst Version 2.0 commercial software, developed by Central Mining Research Institute, Nagpur Centre, in collaboration with Wavelet Group, Pune, India, was used. Although a wide range of image-analysis software is commercially available, ie Wipfrag, Split, Tucip etc, Fragalyst has been well proven under Indian conditions.
Results and discussion
As is evident from the observations provided in table 3, two blasts with hole diameters of 269mm and two blasts with hole diameters of 259mm were studied. A perusal of table 3 indicates that blast nos. 1, 2, 3 and 4 were conducted at stiffness ratios of 2.0, 2.6, 2.9 and 3.4, respectively. The variation in the stiffness ratio could be studied in the field because of variations in the height of the same bench at different locations. Furthermore, all four blasts were drilled in a straight rectangular pattern but fired diagonally. A representative drilling and firing pattern for blast no. 1 is shown in figure 2. The notation 0/100/125/150 in figure 2 and table 3 signifies that the initiation point (IP) is represented by digit ‘0’ and the subsequent delay interval between the diagonal firing rows is 100ms, 125ms and 150ms. Blast nos. 3 and 4 were fired with greater number of firing rows (15 and 14 respectively) in comparison to blast nos. 1 and 2 (11 and 12 respectively). Barring these few exceptions, which were almost inevitable under the field conditions, all the blasts have been assumed to be more or less equivalent from the viewpoint of blast geometry, rock and explosive parameters. Hence,
the comparative fragmentation study of the performance of the blasts, made on the basis of the results obtained from image analysis of the blasted material, could adequately reveal the impact of stiffness ratio (H/Be) alone.
Looking at table 3 for results of fragment size and its distribution, it is quite evident that with the increase in the stiffness ratio, fragmentation inside the blasted muckpile improves. The results in terms of cumulative fragment size distribution curves, as provided by the software, are illustrated in figures 3, 4, 5 and 6 for blast numbers 1, 2, 3 and 4 respectively.
Field photographs (figs 7, 8 and 9) reveal the occurrence of collar boulders from the back rows and boulders inside the muckpile for blast nos. 1 and 2, whereas the photographs captured on the muckpile of blast nos. 3 and 4 (figs 10, 11 and 12) by and large reveal good fragmentation inside the muckpile with good uniformity. Nevertheless, boulders were observed along the back rows of these blasts
(figs 11and 12).
The impact of a change in the stiffness ratio is clearly evident from the relationship exhibited in figure 13, which reveals that with an increase in the stiffness ratio there is a significant reduction in the fragment size. Furthermore, it also reveals that there is a drastic reduction in the fragment size when the stiffness ratio increases from 2.5 to 3. Beyond a stiffness ratio of 3, the curve is observed to be almost flat. The findings are in line with earlier work reported by Konya3.
Smith13 also reported that an increase in bench height for a given burden makes the bench more flexible, giving better fragmentation. Lundborg et al.1 mentioned that a smaller stiffness ratio implies greater stiffness, which, in turn, offers greater resistance to breakage in bench blasts. The poor breakage along the collar region may be attributed to the increased bench stiffness5.
Sarathy14 also reported that a bench height-to-burden ratio of 2.5 to 3 provides the ideal situation for fragmentation as smaller values than this result in a ‘cratering’ effect, which generates flyrock, airblast, vibrations and oversizes.
Conclusion
Based on this case study, it can be concluded that for moderately hard, medium-to-fine-grained sandstone benches, stiffness ratio exerts considerable impact on fragmentation.
For such benches the fragment size first decreases and then increases with an increase in stiffness ratio. The point of inflexion corresponds to a magnitude of almost 3, which means that for moderately high, medium-to-fine-grained sandstone benches, optimum results in terms of fragment size and distribution are obtained at stiffness ratio of almost 3.
Acknowledgements
The authors would like to express their gratitude for the assistance and co-operation of staff, officers and management of Northern Coalfields Ltd.
References
- LUNDBORG, N., PERSSON, P.R., PETERSON, A.L., and R. HOLMBERG: ‘Keeping a lid on flyrock in open-pit blasting’, Engrg. & Min. Jour., 1975, May issue.
- OUCHTERLONY, F.: ‘Review of rock blasting and explosives engineering research at Sve De Fo’, Procs. Explo-95, Brisbane, 1995, pp133–146.
- KONYA, C.J.: Blast Design, pub. by Intercontinental Development Corporation, Ohio 44064, USA, 1995.
- SARATHY, M.O.: ‘Optimum blasting in surface mines – Major issues’, Procs. Sem. on Blasting Objectives and Risk Management, Hyderabad, 13–14 July 2000, pp1–29.
- RAI, P.: ‘Evaluation of the effects of some blast design parameters on fragmentation in opencast mines’, Ph.D. Thesis, 2002.
- JIMENO, C.L., JIMENO, E.L., and F.J.A. CARCEDO: Drilling and Blasting of Rocks, A. A. Balkema, Rotterdam, The Netherlands, 1995.
- MAERZ, N.H., FRANKLIN, J.A., ROTHENBURG, L., and D.L. COURSEN: ‘Measurement of rock fragmentation by digital photo analysis’, 5th Int. Congr. Int. Soc. Rock Mech., 1987, pp687–692.
- MAERZ, N.H.: ‘Photo analysis of rock fabric’, Ph.D. thesis, Dept. of Earth Sciences, Univ. of Waterloo, 1990.
- PALEY, N.L.: ‘Image-based fragmentation assessment’, M.Sc. thesis, The Univ. of Qld, Brisbane, 1990.
- EXADAKTYLOS, G.E., and C.E. TSOUTRELIS: ‘Fragmentation analysis using the photographic method’, Int. Jour. of Sur. Min. & Reclamation, 1991, vol. 5, pp55–64.
- PALANGIO, T.C., and J.A. FRANKLIN: ‘Practical guidelines for lighting and photography’, Procs. Fragblast 5, Montreal, Canada, 1996, pp111–114.
- SCOTT, A., COCKER, A., DJORDJEVIC, N., HIGGINS, M., LA ROSA, D., SARMA, K.S., and R. WEDMAIER: ‘Open-pit blast-design analysis and optimisation’, JKMRC monograph series in Mining and Mineral Processing, Eds. A. Scott and T.J. Napier, Munn, 1996.
- SMITH, N.S.: ‘Burden rock stiffness and its effects on fragmentation in bench blasting’, Ph.D. thesis, Univ. of Missouri, USA, 1976.
- SARATHY, M.O.: ‘Delay blasting – An inexpensive tool for reduced total mining costs’, The Ind. Min. & Engrg. Jour., 1991, Oct. issue, pp51–58.