The Versatility Of Concrete
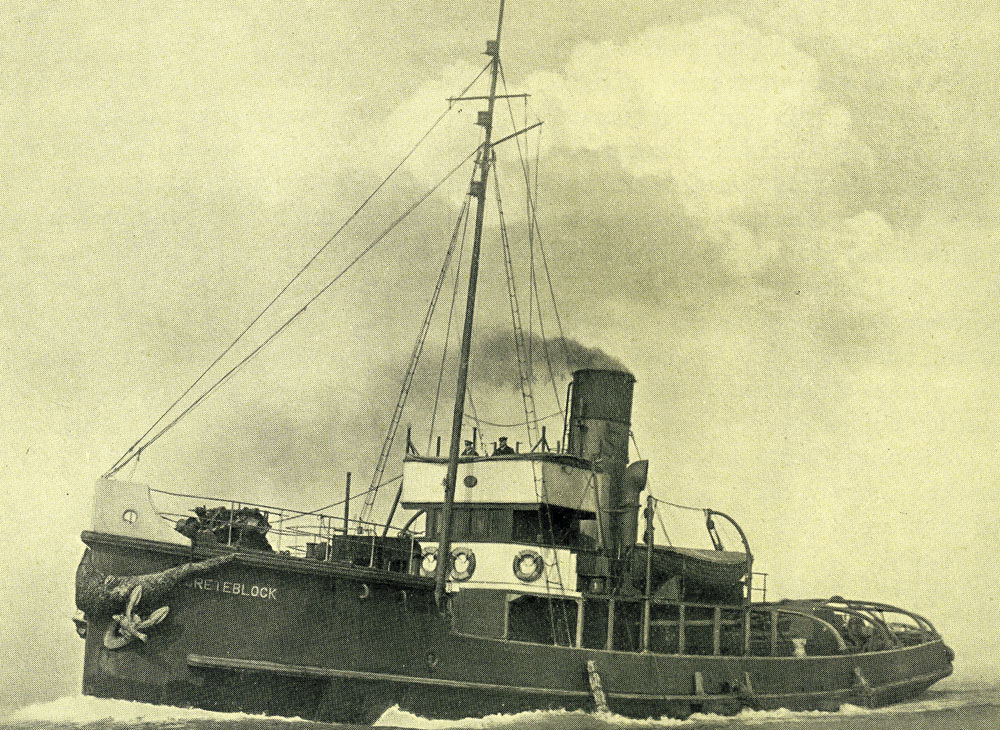
Taking the versatility of concrete to mean its ‘adaptability to a wide variety of purposes’, this paper looks at what it is about concrete that lends itself to varied uses and how those many uses reflect concrete’s character
By Edwin A.R. Trout, manager of Information Services, The Concrete Society
That there are many and varied uses for concrete is indicated by the ‘Little book of concrete’1, a marketing document issued by British Precast around four years ago, which sets out 100 advantages gained by using concrete. In the introduction its compiler writes of concrete: It is the most commonly used building material in the world; yet we take what it does for granted – too often this means that much of what concrete can offer is overlooked.
Concrete’s undoubted versatility has been a gift to those responsible for its marketing over the years. As the author of this paper works for the Concrete Society, running an information service that started out as the library of the Cement & Concrete Association, it begins by looking at some examples of how concrete’s versatility has been promoted over the years by these organizations.
Concrete & Constructional Engineering, the forerunner of the Concrete Society’s Concrete magazine, was launched in 1906. Its editor, the energetic architect Edwin Sachs, was determined to win new markets for concrete: As a pioneer journal on the application of concrete and steel in the British Empire, it is hoped that its pages will not only be of interest and of use to those already directly and indirectly concerned with the subject under review, but that by their advocacy of what is practical and economical in civil and architectural engineering, they will also compel the attention of those who may still be holding aloof from the application of the modern methods of construction dealt with. In fact, this journal should stimulate interest in all appertaining to concrete and steel, and especially in questions relating to their application economically, rapidly and safely2.
The key phrase above is: ‘economically, rapidly and safely’. These were attributes that concrete was seen to offer the construction industry. From the very first issue, in a column headed ‘New Uses for Concrete’, the journal drew attention to how concrete could be, and was being, used around the world.
In 1933 the Concrete Society’s immediate predecessor was established; the Reinforced Concrete Association quickly issued the first in a series of Reviews. No.1 reflected on the progress of concrete in the UK: It is 30 years since reinforced concrete was first used in this country, and during that comparatively brief space of time it has steadily advanced to a leading position among the materials of construction. …For nearly every class of engineering structure, it has become the standard form of construction and the revision of the Building Acts and bye-laws which is now in progress, will render it even more economical and increase its scope for architectural work. In the near future it will undoubtedly be used to as great an extent in this country as on the Continent and in America.
Reinforced concrete has been used for the construction of buildings of every possible type, and future publications in this series will contain illustrations and descriptions of – churches, cinemas, concert halls, dwelling houses, factories, flats, garages, hospitals, hotels, offices, schools, theatres, warehouses etc3.
Only two years later, in 1935, the Cement & Concrete Association (C&CA) was founded and its promotional magazine, Concrete Quarterly, was launched after the War in July 1947. The first issue alone featured dams and coastal defences; bridges, railways, motorways and bus garages; collieries and power stations; water treatment and sewage works; farms; and houses and flats. Subsequent issues included: stadia and swimming pools; churches and prisons; docks, water towers and silos, among many others.
The sheer variety of building types and construction sectors is enormous, and far wider than those for which competitive structural materials are used. Each rival material has its natural uses, where it is employed to optimum effect, but in structural terms the compass of most is relatively limited. Where else would asphalt be used except for paving? Glass is used for windows in all classes of buildings and as cladding in commercial buildings, and though there have been recent moves to use glass structurally, eg in bridges, this remains highly exceptional. Clay is widely used for roofing and wall tiles, and in this country is dominant in masonry, particularly in the residential sector. Timber is used extensively for floors, partitions and cladding, with an increasing role in frames, most noticeably in housing and temporary buildings. Steel is, perhaps, the closest comparable, arising, like concrete, in the later 19th century and competing for the attention of engineers and architects. It dominates in frames for multi-storey buildings; is widely used for floors, roofing and cladding for commercial and industrial buildings; and is extensively used for bridges, silos, gantries etc.
What is it about concrete that allows it to compete in so many markets and to be used in so many ways? What are the characteristics – both those inherent to the material and those imparted by decades of development – that have led to such a wide variety of uses over the years?
Perhaps most fundamental is its plasticity and the variability of its constituents. Unlike any of its rivals, it can be formed into its final shape on site or at the factory. What other material can offer this flexibility? Plastics, iron and steel, glass, and clay products are all produced in the factory or foundry, in the glass-house or brick kiln. Manufactured products are brought to site and assembled as components of the building. Asphalt, conversely, is laid only in situ. Timber, perhaps, lends itself both to prefabrication and to cutting or carving on site. But concrete alone can be moulded into its final form on site as well as in the factory, and that opens up many more options for construction – the method of placing, size of element possible, flexibility of form, adjustability of construction programme – though it does leave concrete vulnerable to charges of inconsistency and difficulties in quality control.
There is also the composition of concrete. To use a well-worn analogy, concrete technology is similar to cake-baking – a wide variety of results can be achieved by mixing a small number of basic ingredients in varying proportions (eg flour, fat and sugar), choosing a binder (eg eggs or milk), and combining extras for special effects (eg fruit, spice, chocolate, essence or food colouring). A sticky mixture is usually moulded to the required shape and a change of state takes place in the presence of heat. The resulting material can then be embellished with a range of surface treatments if desired (icing, chopped nuts etc). Victoria sandwich is conspicuously different from Dundee loaf, but the two are identifiably cakes.
This mixing of varying constituent materials affects its character and allows the basics of concrete to be adapted to innumerable purposes. Some of these characteristics vary little and are common to most concretes. The next section looks at some of these basic attributes, and their influence on early applications, before moving on to look at how concrete has specialized in different directions over the years.
Some inherent characteristics of plain concrete
Hydraulicity
Cement is concrete’s active binding constituent, and the principal cement of the past 170 years – what commentators such as Winn and Howe in the early years of the 20th century have called the ‘Concrete Age’ – is Portland cement. However, there have been other cements during that period and certainly there are many cementitious materials on the market today, but the development of an artificial hydraulic cement capable of industrial production was the aim of cement pioneers from Smeaton to Aspdin. The hydraulicity of a proportioned mixture of chalk and mud was cement’s key attribute: when hydrated it hardened and strengthened, rather than slaked or dispersed. Concrete made with Portland cement could be placed effectively in water. It should be no surprise, therefore, that early applications included harbour works at Dover and Cherbourg (1848–53), then at Le Havre, Brest and La Rochelle.
Adhesion
The power to stick is also a function of the cement content. During the formative years of the British cement industry, the comparative testing of different types of cement was a preoccupation. Portland’s superiority over Roman cement, for instance, was demonstrated in a series of published tests by John Grant in the 1860s. This adhesive quality permits the continuous pours that characterize concrete construction and allow it to combine with other materials. The development of cements has constantly progressed and today there are high-strength specialist compounds, such as Densit’s CRC, which, since the European Concrete Building Project at Cardington, has acted as the ‘glue’ for bonding precast elements.
Plasticity
The consistence or workability of concrete in its plastic state can lie at any point on a continuum from a flowable slurry to a stiff mix before reaching solidity. Its plasticity allows the concrete not only to be moulded, but also affects the method of production and placement. A stiff concrete can be simply tipped down into an excavation, but not if the destination is remote, inaccessible or above the point of discharge from the mixer. The workability of concrete has allowed for increasing mechanical means of placement, eg pumping or spraying.
The cement gun, as it was originally known, used for spraying concrete, was developed in America in 1911 where it had found a niche role in, among other things, constructing concrete bodies for railway wagons immediately after the First World War (fig. 1). Sprayed concrete was introduced to Britain in 1919.
As concrete mixed for casting on site has a limited period in which to be discharged, the mechanics of production are such that concrete can be mixed at a distance, in transit, or on site, in a large static batching plant or in a wheelbarrow. Mobile mixing of concrete has a long history from the Victorian horse-drawn mixer and inter-War concrete train, to the ready-mixed concrete industry of today.
Formlessness or fluidity of form
One of the defining characteristics of concrete is the lack of its own form. It takes up the shape into which it is moulded while in its plastic state. It is cast – pre- or in situ – into a mould or formwork, or a void to be filled. Whereas cement found its initial uses in mortar or stucco applied to brickwork, early concrete took the form left for it in the ground as foundations, or as masonry backing or infill for civil engineering projects. Volume-produced 40-ton blocks for Admiralty breakwaters constituted an early example of precast manufacture, as did the work of the London house builder W.H. Lascelles, but shuttering for in-situ building and bridge construction also developed in the final three decades of the 19th century. Perhaps the most ubiquitous early application of concrete’s ‘mouldability’ was in architectural mouldings: cast stone door and window dressings, string-courses, coping stones etc (fig. 2).
At one end of the spectrum, concrete is often used simply for filling voids that are out of sight in operations such as mine stabilization, an example of which, at Comb Down in Bath, has received publicity lately in the magazine Geotechnical Engineering. On a smaller scale, and a less familiar instance of void filling, is concrete’s former role in ‘tree dentistry’, where for years concrete was used in arboreal circles for plugging hollow trunks and repairing damage to branches.
At the other end of the spectrum is the use of concrete for sculpture, where shape is all-important and structure, for the most part, is not the prime consideration. James Aspdin’s ‘Prophet Samuel’ is an early illustration dating from the 1840s. It served little purpose other than to demonstrate the material, and the illustrative nature of concrete sculpture has been exploited by the promotional literature ever since. Incidentally, the world’s tallest free-standing concrete statue is in Stalingrad: a propagandist celebration of the Soviet Union’s victory over the German army in 1942. A symbol of the ‘motherland’, this sword-wielding female figure stands over 80m tall – the equivalent of a 30-storey building.
Compressive strength
‘Concrete is strong in compression but weak in tension’. How often is that heard? Yet this is, without doubt, one of the fundamental characteristics of concrete. Why else would concrete have so quickly found a niche as the foundation material par excellence and become so closely associated with the requirements of civil engineering? As E. Dobson said as early as 1850: ‘Concrete is a valuable material when applied in a proper manner, viz. in underground works where it is confined on all sides, and is, consequently, subjected to little cross strain’4.
The strength conferred on concrete by the choice of cement was a matter of constant experimentation in the 19th century, and Portland cement’s success over rivals such as ‘Roman’ and ‘British’ cement was largely down to strength. Concrete’s strength was celebrated in the building materials section of the Great Exhibition in 1851, where several concrete products were tested to destruction.
In the decades following the Exhibition concrete was used in its plain or mass form, for docks, foundations, dams etc, applications that required the bearing of compressive loads.
Weight
Concrete is inherently heavy, consisting largely of aggregate derived from stone – be it crushed rock or gravel. Often this is a desirable quality in providing solidity and resistance to movement, eg in early coastal defences. Concrete has been used consciously as a weight, often a counterweight, but the greater challenge has been to find ways of lessening the weight of concrete, of developing a lightweight aggregate that does not necessitate the sacrifice of strength. This will be looked at later in this paper.
Toughness and impact-resistance
In its resistance against rough treatment, impact and physical damage, concrete has had few challenges more severe than in its military use as a defence against projectiles and explosive munitions: bullet-proof concrete, bomb-proof concrete and missile-proof concrete. There are many early and recent examples.
During the late 19th and early 20th centuries massive systems of fortification were built in Europe: Dutch forts along the German border, bunkers in the trench systems of the Western Front, the Siegfried Line, the Maginot Line. Britain fortified its south-coast naval installations and imperial stations such as Hong Kong and Singapore. Indeed, much of the pioneering research into cement and concrete in this country was conduced by the military and naval establishments at Chatham.
As the Second World War approached the threat from mass bombing was recognized and the British Government planned for bomb shelters. Concrete Publications Ltd issued Trench, surface, bomb-proof and other air-raid shelters in 1939, written the young Ove Arup. As the war unfolded pillboxes, anti-tank defences and gun emplacements followed. On 23 March 1942 Hitler ordered the construction of the Atlantic Wall, a huge undertaking of fortification from northern Norway to the Spanish border, 10% of the concrete for which was allocated to fortifying the Channel Islands. Towards the end of the war the German Wehrmacht prepared to conduct an air campaign against London by launching V2 rockets from a secret bombproof bunker in northern France. In Calais, a colossal dome, 72m in diameter and seven storeys tall, was erected to construct and house the rockets prior to launch. Some 55,000 tonnes of heavily reinforced concrete were used, 5m thick, and designed to be impregnable against aerial bombardment. It was captured by Allied troops before it had become operational.
Armament and protection have since continued, as always, to leapfrog each other in development. During the Gulf War the GBU-028 ‘Bunker Buster’ bomb was developed that would penetrate up to 7m of concrete. Now the Americans have developed a form of very high-strength, steel-fibre-reinforced concrete with the intention of replacing hardened steel plate armour. With a tensile strength twice that of conventional concrete, it will resist most forms of ammunition. On a more modest scale, Phil Purnell at Leeds University has recently proposed a development of cementitious composite discs to be used in bullet-proof vest manufacture.
Massiveness
Concrete’s weight, strength and robustness add to the monolithic nature of the material. In its early applications concrete was often a ‘massive’ material, most characteristically used in infrastructure projects and on a big, occasionally monumental scale.
Looking backwards, the first issue of Concrete & Constructional Engineering in 1906 traced progress from dock walls to foundations and reservoirs, from ‘earlier massive works into building and into bridges especially’. The railways had become ambassadors for concrete, most notably the North British Railway on the line from Fort William to Mallaig. Along this route there were retaining walls, platforms and station buildings, bridges and viaducts – especially the Glenfinnan Viaduct, the acme of contemporary construction in mass concrete. About the same time the newly combined Associated Portland Cement Manufacturers (later Blue Circle Industries) issued a glossy promotional book entitled A Great British Industry. It highlighted the major projects in which the company’s products had featured over the previous years. Perhaps predictably they comprise big civil engineering structures, as shown in table 1.
Table 1
Date - Project
1855 - Tilbury Docks
1895 - Southampton Graving Dock
1879-1881 - New Eddystone Lighthouse
1883-1887 - The Tay Bridge
1892-1897 - Blackwall Tunnel
1881-1888 - Vyrnwy Dam
1898-1902 - Assouan Dam
1904 - Frankley Reservoir, Birmingham and corporation waterworks
Durability
Durability is taken to mean resistance to internal and external deteriorative processes, to long-term degradation. This aspect of concrete is of ongoing interest and there is an immense amount of literature on it, but for the purposes of this article it is suffice to say that a measure of concrete’s durability is the state of repair of early concrete structures. The Pantheon of ancient Rome is the obvious example, even the great pyramids of Egypt according to some theories, but this paper takes a look at a form of concrete more akin to present-day Portland cement concretes and looks at its long-term performance in highly aggressive, marine conditions. The concrete ships built at the end of the First World War form a readily-identifiable corpus of examples of concrete structures subject to torsional forces at sea, impact from collision and the effects of corrosion from saltwater.
As the RCA’s Review of September 1936 pointed out, ‘it would be difficult to devise a severer or practical test of the durability of a structural material than to build seagoing ships with it, and put them to work under such conditions as have been encountered by the Creteboom, Cretestem, Askelad and Polias’. The former, after 15 years’ service in the ice-bound Baltic, though no longer of attractive appearance, was described as ‘perfectly sound’. In 1922 a salvage survey of Polias, sunk two years earlier, claimed ‘the test is one showing very clearly the high resistance of reinforced concrete to exceptionally severe shocks and stresses’, contrasting the ship’s condition with a steel or timber vessel which ‘would not have survived for more than three months in the same situation’. The Review was ‘written with the object of demonstrating the durability of reinforced concrete as a structural material’; a more scientific and systematic survey, entitled ‘Concrete in the oceans’, was conducted by the C&CA and partner organizations in the 1970s and 80s.
Today, it is well known that durability is a more complex issue than was considered to be the case in the 1930s. For example, in recent years bridges with a nominal design life of 100 years have been examined for the deleterious effects of ASR and thaumasite, but there are many examples of 19th century bridges extant, such as the very early Flixton Bridge of 1870.
The Hoover Dam was built in the 1930s, supposedly with a design life of 2,000 years, though there will be a long wait to see if it outlasts the Pantheon’s present-day achievement. Austrian inventor Ludwig Hatschek was even more confident in the durability of fibre cement when, in 1900, he named his new product Eternit, from the Latin ‘aeternus’, or everlasting.
Fire resistance
One of concrete’s early claims to special attention was its fire-resistant quality (fig. 3). After a vogue for cast iron floors, the first genuinely fire-resisting floor was patented by Dr Henry Hawes Fox in 1833. It contained a lime concrete. Other designs followed and by the 1880s so-called ‘clinker concrete’ was widely used in conjunction with iron joists as a means of constructing fire-resistant floors in commercial and public buildings.
A series of disastrous fires in London theatres during the early 1890s motivated the young architect and architectural historian Edwin Sachs to devote his professional energies to improving theatre design and seeking the best methods of fire prevention. He established the British Fire Prevention Committee, forerunner of the Fire Research Station (now part of BRE) and organized the International Fire Prevention Congress in 1903. Significantly, after a series of BFPC investigations, he came to the conclusion that the new material then being introduced to the British market, reinforced concrete, would be the best for constructing fire-resistant buildings. It was this aspect of concrete’s performance that enthused Sachs in its favour and prompted him to found the journal Concrete & Constructional Engineering in 1906 and the Concrete Institute (later the Institute of Structural Engineers) in 1908.
In the 1930s the Reinforced Concrete Association was quick to promote ‘resistance to fire’, featuring the topic in its fifth promotional Review and pointing to an early example of an entire building subject to fire – the Edison Phonograph Works in New Jersey. On 19 December 1914, this factory, packed with gramophones, records, films and projectors made of highly combustible wax and celluloid, succumbed to a conflagration of great intensity. An international investigation followed, comparing the traditional brick structures with the new reinforced concrete. Whereas the traditional buildings were destroyed, the concrete structures were returned to manufacturing within 30 days.
Fire resistance remains one of concrete’s claims, though there has been some dispute with the promoters of steel in recent times over the nature of such comparisons.
Characteristics of concrete after the advent of reinforcement
The urgency of finding a suitably fire-resistant building material in the 1890s coincided with the scientific development of reinforced concrete, largely by French and German-speaking pioneers. With the advent of commercially promoted reinforcement in Britain from 1897, the nature of concrete, and the range of its corresponding applications, underwent a huge change. An enormous number of new possibilities were opened up for exploitation by the new material, enthusiastically promoted by the Fire Prevention Committee, the RIBA and the newly established Concrete Institute. Looking forward at the dawn of the ‘Concrete Age’, the prospects for the recently patented systems of reinforced concrete were waiting to be realized.
Tensile strength
The juxtaposing of concrete’s resistance to compression with steel’s resistance to tensile stresses was fundamental to reinforced concrete’s success, and one of the earliest British writers on reinforced concrete, Lt Col J. Winn RE, was quick to point out the opportunities in 1906: ‘the introduction of steel to enhance tensile strength extended concrete’s employment into new elements: floors, beams, arches, piles, walls, partitions, pipes, aqueducts, bridges of large spans, tall chimneys, lighthouses. …Immense warehouses, depots, cold storage sheds, drill halls, and factories have been built…entirely of this reinforced concrete. …It would be impossible in the space of a short article to refer to all the varied uses to which reinforced concrete has been applied’.5
Francis Onderdonk, an advocate of architectural concrete writing in the 1920s, looked back to the origins of reinforcement: ‘In 1878 Monier claimed for reinforced concrete that, as far as form is concerned, it has no limits. Reinforced concrete has conquered the third dimension’.6
He went on to write: ‘Reinforced concrete combines the advantages of steel, stone, and wood, eliminating many of the disadvantages of each. The reinforcing bars, bendable and not limited as to length and slenderness, can follow the most complex lines and curves and give the surrounding concrete an indestructible backbone. Being plastic when placed, concrete can adopt any conceivable form. Practically unlimited length, slenderness, and intricacy are combined with strength and durability in concrete’.
Purposely to illustrate concrete’s strength, and in a gesture reminiscent of the Great Exhibition of 1851, the organizers of the Cologne Werkbund exhibition in 1914 had a structure erected where a tower surmounted three nearly horizontal supports over a void below (fig. 4).
The later development of pre-stressing accentuated concrete’s performance in tension, making longer-span roofs and bridges possible, and more recently still, the makers of the proprietary material Ductal have imparted greater tensile properties to the concrete itself.
Monolithicity
The monolithic nature of the material allowed a new structural continuity of form. Elements could blend seamlessly, and whereas the structural use of steel and timber for spans depended on a framework of beams, girders, struts and rafters, reinforced concrete was able to incorporate the slab itself as a structural element. In buildings, flat-slab construction developed from C.A.P. Turner’s mushroom-head system first used in 1904, and in bridges, integral deck slabs or ‘beamless decks’ were designed by R. Maillart from 1910.
Again contrasting with steel, concrete could adopt curved organic shapes that were otherwise achieved in the vertical plane by brick or on a small scale by mud or plaster construction. Concrete could appear to flow in three dimensions. The extraordinary Einstein Tower at Potsdam (fig. 5) is a good example of a building that appears to have been moulded, while in contemporary architecture, exponents such as Zaha Hadid have acquired reputations for the organic sinuousness of their designs.
Slenderness
Reinforcement allowed a slenderness of form and a gracefulness of construction not possible with plain concrete. Massiveness was increasingly replaced by slenderness, a trend that has continued with the development of pre-stressing, higher-strength concretes and glass-fibre reinforcement.
Such slenderness found expression in the roofs that were now possible over ever-increasing spans. The archetypal form was the shell roof, a form that emerged in Germany before the Great War. It reached its apogee in the work of Nervi in Italy and Candela in Mexico. A dome roof was designed in 1917 at the London offices of Christiani & Neilsen, but in this country shell roofs generally had to wait for the era of pre-stressing that boomed in the 20 years or so from 1945.
In the case of church-building, where elegance and visual appeal have long been valued, the contrast was marked by, for example, the architect Auguste Perret who introduced concrete tracery in the 1920s to create interiors of lightness and delicacy, complementing his soaring church towers (fig. 6).
Architectural use
New structural forms allowed a new aesthetic, and although architects in this country did not hasten to explore concrete’s potential as a new medium, by the 1920s the work of Sir Maxwell Ayton and Owen Williams was prompting interest. In 1927 Bennett & Yerbury’s photographic survey ‘Architectural design in concrete’ promoted the idea of a new concrete architecture, where form reflected purpose, construction was monolithic, and wide spans, cantilevers, slender columns and continuity of support throughout the height of the building was possible.
A year later, Francis Onderdonk’s pedagogic book, Ferro-Concrete Style, more emphatically advocated a style of architecture specific to concrete, pointing to its spontaneous, if uneven, emergence in Europe and America. He quoted Dr A. Willnow’s claim that ‘Ferro-concrete, in contrast to steel, is capable of creating an architecture’. A sweeping claim indeed, but looking at the impact of new materials, one that Bennett had also made. Similarly, R.J.M. Sutherland has since suggested that ‘concrete was ideally suited to the post-war fashion for ‘functional’ architecture. It could even be argued that the fashion was largely created by the material’.7 The symbiosis of steel and concrete allowed reinforced concrete construction to take on new structural forms and, combined with concrete’s freedom of shape, allowed the development of a distinctive new architecture.
Onderdonk quotes a florid statement by the architect J.J. Earley: Works great and small, well done with concrete, will accumulate day by day until the prestige of concrete has developed power enough to sweep away all that is in the way of its natural advancement to its place in architecture and in art as the most satisfying medium which has yet been devised.
Key to Onderdonk’s thesis was the centrality of the parabolic arch in the new structural design, the use of tracery, and the possibilities of integral colour and surface texture.
Coloured and textured finishes
The possibilities of colour and texture were explored by Onderdonk with some enthusiasm. The plates in his book are a testament to the vividness of effect made possible by coloured aggregate and the various finishing treatments he espoused, perhaps most notably at the Shrine of the Sacred Heart in Washington, DC.
Concrete may naturally be a shade of grey, and this fact has left a negative and perhaps indelible impression on public perceptions of the material. It certainly need not be that way, however, as the producers of pigments have endeavoured to prove and the vibrant work of artists such as Carole Vincent and David Undery, captured on the pages of Concrete Quarterly, has shown in recent years.
Lightness
Combating the innate heaviness of concrete was a challenge that confronted the promoters of concrete in the early years of the 20th century. The American Stephen J. Hayde is known as the father of lightweight concrete, having developed the first commercially viable manufactured lightweight aggregate. A brick-maker by trade, and a contractor, Hayde endeavoured to find a use for the waste ‘bloaters’ from his brickworks and in 1897 considered crushing them for incorporation in concrete. Bloaters were bricks that had expanded excessively in the heat of the kiln, resulting in a structure of internal voids encapsulated by a hard ceramic matrix. They were lightweight and when crushed had no plane of weakness. Years of observation and experiment led to a patent for ‘Haydite’, filed in 1914, then practical trials in Kansas City showed that a rotary kiln could be used economically to produce expanded clay or shale aggregate. In 1917 he was confident of the technology and after America entered the First World War he wrote to the newly established Department of Concrete Ship Construction offering free use of his invention for the duration. Thus the first application of lightweight concrete with manufactured aggregate was in the ships of the US Emergency Fleet.
The first of such were the 3,000-ton Atlantis and the 7,500-ton Selma. The Department, having calculated that a large vessel would not be feasible unless the concrete had a compressive strength of ‘500 lb/in2’, and a weight of ‘no more than 110 lb/ft3’, had embarked on similar experiments with expanded slate, clay and shale. Atlantis was built with the output of these trials, and Selma with the superior Haydite.
With the coming of peace new uses were sought and the 1920s in America saw the application of lightweight concrete to high-rise building. Most celebrated was the extension of the 14-storey Southwestern Bell Telephone Co. building in Kansas to twice its height, compared with the additional eight floors possible with normal-weight concrete.
Since that time, Siporex and autoclaving, and newer types of aggregate, have provided alternative ways of achieving reductions in weight.
Water-resistance
Concrete is inherently permeable, but the degree of porosity varies greatly and it is notable that several of its major early applications were for water-retaining structures: docks, the London sewers, dams, and with the advent of reinforcement, tanks and water towers. The first concrete water tower in Britain was at Mayrick Park, Bournemouth, in 1900, to a design that set the standard for many years to come. It was followed in 1904 by the enormous tower at Newton-le-Willows, which, for a time, was the largest in the world. These and their numerous successors imposed their superiority on a water supply industry that, throughout the 19th century, had relied on sectional tanks made from cast iron panels.
Public baths and swimming pools had emerged as a feature of urban life in the Victorian years, but it was in the 1930s – the era of the ‘lido’ – that pool construction really took off, vigorously promoted by the Amateur Swimming Association (ASA). The material of choice was concrete – and not just for the pool itself, but the structure of the building surrounding it (fig. 7). The ASA’s seminal book on modern public baths in 1938 trumpeted the association’s achievements in promoting a new architecture for this purpose.8
The 1930s saw a renewed interest in water-retaining structures generally – reservoirs, tanks and water towers – prompted and served by W.S. Gray’s books on these subjects in 1931 and 1933. Revisions, later known as Gray & Manning, continued to be issued by Concrete Publications Ltd and the C&CA into the 1970s.
Drainage has seen the role of concrete diverge to serve two extremes: large-diameter pipes to serve the supply of water, and porous concrete to allow controlled surface drainage. Although ‘no-fines’ concrete has been used to control highway run-off for many ears, the widespread flooding of 2007 has given an additional urgency to the now fashionable ‘SUDS’ and provided a new market opportunity for the concrete block paving industry. In America, a parallel interest in ‘pervious concrete’ is receiving a lot of attention in the technical press at present.
Acoustics
Ever since Thomas Edison’s experiments with a concrete piano, the acoustic qualities of concrete have been exploited. One of the more iconic uses of the material during wartime was the listening station on England’s south coast for which giant ‘ears’, or ‘listening mirrors’, were built of concrete. In more recent times concrete’s greater resistance to sound than that of rival lightweight materials has been promoted for multi-storey residential construction and for compliance to Part E of the Building Regulations. Concrete barriers to protect against noise have been built along motorways and at airports, such as the distinctive wavy acoustic wall at Gatwick. At the opposite end of the scale, bespoke concrete casings for hi-fi systems have been heavily featured in the press recently.
Density
The density of concrete varies from the open textures of foamed or aerated concrete to that suitable for radiation shielding. Originally for defensive military purposes in the Cold War, against the threat of nuclear attack, concrete with heavy natural aggregates such as barites or magnetite is now often used in medical practice.
Fibre reinforcement
In parallel to reinforced concrete, the development of fibrecement in 1900, while perhaps not strictly concrete, provided a cementitious alternative that had its own distinctive range of characteristics, many of them opposite to those of reinforced concrete. Slender, lightweight and water resistant, fibrecement products came to prominence in the roofing and drainage markets.
Enhanced properties of concrete in more recent times
At the time of Concrete Quarterly’s launch in 1947, much of the technology associated with concrete construction was recognizably mature. Concrete was available as plain, reinforced and pre-stressed; the concrete industry was structured to provide ready-mixed concrete and had developed a wide range of precast products. There were, of course, many further developments to come and during the following boom years the scale of concrete construction increased enormously.
Admixtures
During these years the increasing use of chemical admixtures extended the properties of concrete in both the fresh and hardened state. Accelerators, plasticizers, retarders etc, allowed concrete to be used in ways not previously practicable.
In recent years, the application of concrete to new uses has been a result of a conscious extension of the material’s properties. Emerging problems have prompted an exploration of the possibilities of concrete to find innovative solutions, and concrete has been taken in some surprising directions. The motives for such developments lie in the preoccupations of the modern age: a new concern for sustainability and environmental performance; continuing economic advantage; and the avoidance of liabilities in a litigious industry; as well as a timeless desire to seek purely aesthetic enhancement.
Sustainability and environmental responsibility
Some of these developments to enhance the environment have been active innovations in materials or design. In the late 1990s researchers in Japan developed a concrete block containing photocatalytic compounds that captured and dissipated urban pollution. In a similar vein, the coating of concrete in titanium oxide has been found to render concrete self-cleaning and has opened opportunities for low-maintenance facades. Energy efficiency is being improved through the use of concrete floor slabs for integral heating and one manufacturer has developed a paving slab for generating electricity through the action of repeated footfall.
More traditional attributes of concrete have also found a new prominence. Concrete has always had the potential to incorporate recycled material and in the 19th century crushed bricks and coke breeze were widely used as aggregate – even oyster shells and broken crockery in some instances. Indeed, materials that had been through the firing process were thought to enhance concrete’s fire-resistance properties. Since then some recycled materials have been found to be deleterious – mundic is an obvious example – but others have been successfully used for many years, rising in status from waste, to by-product, to construction materials in their own right. In the 21st century the drive to incorporate new streams of waste from demolition sites and industrial processes, such as glass production, has been pursued with considerable vigour and has been widely promoted as one of concrete’s ‘green’ credentials.
Occasionally, this newly revived enthusiasm for encapsulating wastes is taken to unexpected lengths. The Guardian published a piece recently intriguingly referring to ‘dog poo concrete’, in which the German architect Freidrich Lentze was described as believing that all dog waste collected by local authorities should be processed as a construction material: ‘it makes a great mortar with fantastic insulating properties’.
Concrete’s thermal mass has been highlighted by organizations such as the Concrete Centre as one of the benefits that comes with concrete when seeking to regulate the internal temperature of buildings. And the natural process of carbonation, long seen as an issue of durability, has been re-presented as an environmental advantage to partially offset the unavoidable production of carbon dioxide by the manufacture of Portland cement.
Economic advantage
Though there is nothing new in the desire to cut costs and maximize profits, the construction process might be thought of as leaner as a result of changes to concrete technology. New codes of practice, reflecting technical advances, permit concrete to be designed with more slender elements and longer unsupported spans. Concrete’s naturally heavy weight has been reduced, not just by the traditional use of lightweight aggregates, aeration or foaming, but by void-former systems such as Bubbledeck and its equivalents. Systems of reusable formwork, proprietary reinforcement, prefabrication of elements and the use of self-compacting concrete for congested or inaccessible areas have all contributed to the efficiency of concrete construction, of what was once a labour-intensive process.
Aesthetics
But perhaps some of the most eye-catching recent developments in the changing nature of concrete have been in its aesthetic qualities. These, naturally, have lent themselves to visual presentation and have, therefore, featured in the industry’s promotional publications to a greater degree than their use might warrant. However, they do fly the flag for concrete and point to new possibilities that, as haute couture does for high street fashion, might find their way into commercial applications. Their initial value lies in their ability to influence the image of concrete and its perception by the public.
In 2002 the former British Cement Association (BCA) sponsored a competition at the Royal College of Art. The brief was to ‘demonstrate versatility and innovation in the use of cement and concrete’, with the BCA looking for ‘a breakdown of assumptions of cement and concrete as a material through innovative thinking’.9 What better cue for pursuing the theme of this paper into recent times. Entrants displayed a wide range of artistic and technological ideas to various architectural, sculptural and interior design purposes, but several themes emerged. Together, they provide a snapshot of progressive thinking about how this versatile material can be applied in unexpected ways, and reflect some of the underlying trends in concrete technology.
One of the more prominent trends was the combination of concrete with other materials, such as glass and ceramics. Concrete has the ability to bond with or to encapsulate other materials homogeneously, and decorative composites such as Metzzo have been a sideline of concrete for some time. Only last year Lignacite’s architectural masonry block containing coloured glass aggregate was featured as AJ Specificiation’s ‘material of the month’ (January 2010). Not only was the glass recycled glass, but light emitting too, thus combining three modern trends in one.
A combination of materials that has gained particular favour recently is concrete with cloth. The RCA shortlist in the first three years included flock-patterned tiles, a wedding dress with delicate concrete decoration, ‘Concrete Canvas’ cement-impregnated emergency shelters, lacy lampshades, a lattice chair inspired by gossamer veils, and panels cast in the shape of sheets hanging out to dry. Further afield, Queen’s University has been running its ‘Girli Concrete’ project for some time, exploring the relationship between the traditionally ‘masculine’ qualities of concrete with the softer appeal of textiles. The design practice Concrete Blond is designing bespoke, fabric-based concrete interiors, and lace-patterned concrete by Caruso St John features as a thematic motif at the award-winning Contemporary Arts Centre in Nottingham.
In similar vein, concrete and light have been explored by the RCA students with concrete lamp stands and lampshades, the integral use of fibre optics, and the application of solar power to a garden light feature. Likewise, the latest issues of OpusC magazine have featured concrete lamps from studio producers.
Other combinations with concrete have been even more imaginative. One installation consisted of a series of concrete blocks containing computers connected to an online chat room, allowing the blocks seemingly to ‘talk’ to each other. Another depended on the incorporation of magnets to attract and repel.
In some instances the concrete replicated other materials. Perhaps the most successful was an apparently steel and formica table, chipped at the edges and covered with mug rings and coffee stains; it looked so realistically tatty that it was almost disposed of by the cleaners after the exhibition had been set up. Similarly, photographs of a pigmented concrete pouffe (seemingly upholstered in burgundy leather) were recently published in the promotional press, illustrating just how convincing concrete can be at imitating other materials.
The nature of concrete itself was the subject of several competition entries, where external conditions would effect changes in the material. Thus, concrete ‘Weather Tiles’ were designed to change colour in the rain to reveal an image or inscription. Another project caused the concrete to change colour when heated. In a third, concrete street furniture would give off the relaxing scent of lavender when stroked, achieved by the micro-encapsulation of perfumes.
The promotional press loves these innovative, even off-beat adaptations of concrete. Magazines such as OpusC (Germany), Concrete (New Zealand), Concrete Trends (South Africa) and Betoni (Finland) are among the more active in this approach. Other innovations have also graced their pages in recent times, supplementing the RCA projects with eye-catching novelties, each of which pushes the boundaries of what concrete can offer.
One RCA competitor’s method of transferring a photographic image to a mortar-covered canvas reflected a continental trend for photo-etching entire buildings that has been followed by the press over the past decade. Both translucent and light-emitting concrete – Litracon and Luccon – have received publicity well beyond their current levels of use.
But this is the point. Novelties point the way and today’s innovation eventually becomes tomorrow’s tradition. New uses arise continually as the material is adapted to meet the challenges of the future. Concrete continues to demonstrate such versatility that its applications appear unending.
References
- ‘A little book of concrete: a guide to one hundred advantages’, British Precast, Leicester, [c.2007], 115p.
- SACHS, E.O.: Announcement, Concrete & Constructional Engineering, 1906, vol. 1, no. 1, pp.1-6.
- ‘Reinforced concrete buildings’, The Review of the Reinforced Concrete Association, 1933, no.1, pp1-4.
- DOBSON, E.: ‘Foundations and concrete works’, Crosby Lockwood & Co., London, 1850.
- WINN, J.: ‘Advent of the concrete age’, Concrete & Constructional Engineering, 1906, vol. 1, no. 1, pp7-16.
- ONDERDONK F.S.: ‘The ferro-concrete style’, Architectural Book Publishing, New York, 1928, 265p.
- Historic concrete, Proceedings of the Institute of Civil Engineers: Structures and Buildings, Aug/Nov 1996, pp255-480.
- CROSS, K.M.B.: ‘Modern public baths’, London Amateur Swimming Association, 1938, 113p.
- Awards 2002, British Cement Association, Crowthorne, 2002, 13p. (and for 2003 and 2004).
Acknowledgement
This paper was presented at the Institute of Concrete Technology (ICT) annual technical symposium in 2010 and published in the ICT Yearbook 2010-2011. It is reproduced here by kind permission of the ICT.